I’ve written quite a few columns about electricity generation, but until this week have left nuclear power out of the conversation. Given that nuclear power currently generates 14% of the world’s electricity, I thought it was about time for a Common Science review of this fascinating, yet controversial, technology.
With the exception of solar power, electricity is generated through the use of various methods to spin a turbine. (For an explanation of why spinning a turbine generates electricity, see my previous column Electricity Generation 101.) The turbine can be spun directly, with wind or falling water, or indirectly, by directing steam from boiling water through it. Traditional power plants burn coal or natural gas to make steam, while nuclear power plants use the heat from the fission of uranium atoms.
Before exploring fission, let’s start with a quick review of atomic structure. Atoms are made of an equal number of positively-charged protons and negatively-charged electrons, as well as a number of neutrons. Neutrons serve as spacers in the nucleus to keep the protons from getting too close to each other. If protons get too close together, they repel each other in a manner analogous to placing the positive ends of two magnets near one another.
The atomic number of an atom corresponds to the number protons and electrons it has. The atomic mass of an atom is the combined number of protons and neutrons. (We ignore the electrons in this calculation because their mass is negligible.) A complicating factor when discussing atomic mass is that not all atoms of the same element have the same number of neutrons. I’ll use uranium as an example. All uranium atoms have 92 protons. The vast majority of uranium atoms have 146 neutrons, giving them an atomic mass of 238 (92+146). A small fraction of uranium atoms have only 143 neutrons, resulting in an atomic mass of 235. Atoms of the same element with differing numbers of neutrons are called isotopes and referenced by their atomic masses. The two isotopes of uranium are called U-235 and U-238. Since U-235 has fewer neutrons than U-238, its positively-charged protons reside in closer proximity to one another than those in U-238. This makes the nucleus of U-235 somewhat unstable. The relative instability of U-235 is what makes it useful as nuclear fuel.
Uranium deposits contain approximately 99.3% U-238 and 0.7% U-235 and are recovered with standard mining techniques. In order to produce useful nuclear fuel, the U-235 must be separated from the U-238. The purification of U-235 occurs in two steps, both of which have received quite a bit of press over the past decade. First the ore is crushed and treated with acid to recover a uranium-enriched solid known as yellowcake. Yellowcake should sound familiar. In his 2003 State of the Union address, then president Bush announced that the British had determined that Saddam Hussein had been negotiating to purchase yellowcake from Niger. This turned out not to be true.
The next step in the enrichment process is to react the yellowcake with fluorine to make uranium hexafluoride gas. If you run uranium hexafluoride through a centrifuge, you can separate the molecules which have U-235 from those which have U-238 based on the difference in mass. Since the mass difference is extraordinarily small, the centrifuges required are very sophisticated and are considered the most critical piece of equipment in uranium enrichment. Concerns over potential atomic weapons development in Iran often center on centrifuge capability. Once you have separated out the U-235 containing uranium hexafluoride molecules, you can strip the fluorides back off to provide you with nearly pure U-235.
At this point we need a quick Einstein tangent. Everyone knows the equation E = mc2, in which E is energy, m is change in mass and c is the speed of light. When you break an atom apart into smaller pieces, the sum total of the mass of the pieces formed is less than what you started with. If you then multiply the loss in mass by the speed of light squared, you can calculate the energy liberated when splitting the atom. Thinking about the interchangeability of mass and energy sort of hurts my head, so I encourage you to adopt my approach, and just accept it.
OK, let’s get back to our nuclear power story. To generate heat from U-235 you need to break it into smaller pieces. The process begins by firing a neutron at a U-235 nucleus at mind-bogglingly high speed. The neutron is temporarily incorporated into the uranium nucleus creating a new, short lived U-236 isotope. U-236 is unstable and, almost instantly, breaks apart into two smaller atoms, and two or three free neutrons. As discussed in the paragraph above, the combined masses of the pieces created when breaking the U-235 are less than what you started with. The energy liberated corresponds to the change in mass times the speed of light squared and is used generate steam.
If you were reading closely you will have noted that using one neutron to split the U-235 nucleus created 2 or 3 free neutrons. These free neutrons have sufficient energy to break nearby U-235 nuclei. If this process is left unchecked you get a chain reaction, with each fission event initiating 2 or 3 more, which is the mechanism which generates the explosion in an atom bomb. Nuclear reactors, when functioning properly, regulate the rate of fission through the use of control rods. Control rods are made of a variety of metals which are efficient in capturing neutrons without themselves undergoing fission.
The appeal of nuclear power has two origins. First, uranium is a fairly common element on earth so there is an abundant supply. Second, the process does not release green house gases into the air. The number of nuclear reactors around the world grew steadily from the 1960s through 1986 to the current global number of around 450, when disaster struck in Chernobyl. Since then, the rate of construction of new plants has roughly equaled the number that have been retired. As you likely know, the key issues with nuclear power include management of radioactive waste, operational safety, and possible use of enriched uranium for weapons. I’ll deal with those issues in Part II next week.
Have a comment or question? Use the comment interface below or send me an e-mail to commonscience@chapelboro.com.
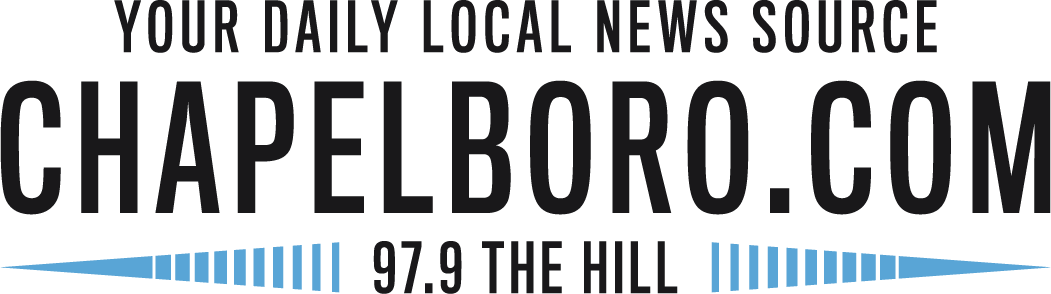
- Listen Live
- News
- Sports
- Town Square
- Newsletters
- 97.9 The Hill WCHL
- Things To Do
- Downtown Shopping Guide
Select Page
Comments on Chapelboro are moderated according to our Community Guidelines